The isotopic composition of natural materials in present-day environments and in the sedimentary rock record can be used to probe the physical conditions, chemical composition, and biological activity of modern and ancient environments.
To this end, we need to understand the process that generates some of the largest stable isotope signals—metabolism.
We pioneered the development of metabolic-isotopic network models, which allow mechanistic understanding of the controls on isotopic fractionation during metabolic activity. These models combine thermodynamics and enzyme-level kinetics to predict the rates of individual reactions in metabolic networks and the associated isotopic fractionations.
In "inward-facing" investigations, we use these metabolic-isotopic models to learn about microbial physiology and evolution. In "outward-facing" investigations, we use them to probe modern and ancient environmental parameters, like the energy available to the microbes in-situ, and the controls on the isotopic composition of natural materials.
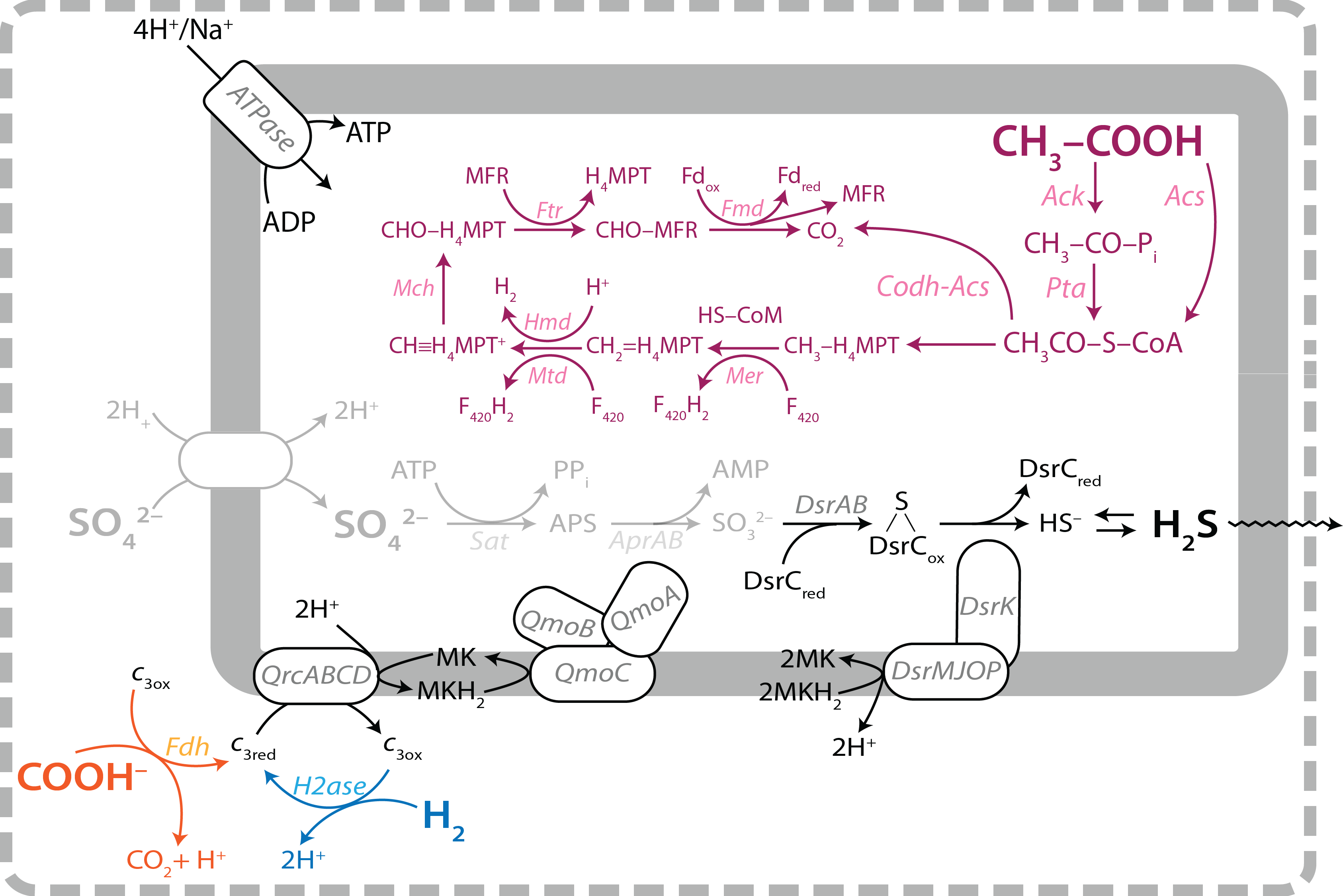
Reactions in the dissimilatory sulfate reduction pathway. The gray reactions have been implemented in our first metabolic-isotopic model (Wing and Halevy, 2014), and the black and color reactions are being implemented in complete models of sulfate reduction. With these additions to the model, we will gain a nuanced understanding of the controls on the fractionation of sulfur and oxygen isotopes in dissimilatory sulfate reduction.
Examples:
We then used this model to constrain the physiological electron carriers used in sulfate reduction, to show that the sulfur isotope fractionation can sometimes reflect the microbes' past rather than present physiology, and to constrain environmental cell densities in-situ.