The quantum twisting microscope
Researchers from the Department of Condensed Matter Physics have developed a new tool—the quantum twisting microscope (QTM)—that can create novel quantum materials while simultaneously gazing into the most fundamental quantum nature of their electrons. The team’s findings, published in Nature in 2023, may be used to create electronic materials with unprecedented functionalities.
The QTM involves the rotation (“twisting”) of two atomically thin layers of material with respect to one another. The angle of the twist is the most critical parameter for controlling the behavior of electrons in the material: Changing it by merely one-tenth of a degree could transform the material from an exotic superconductor into an unconventional insulator. Controlling the twist angle is the biggest challenge to making this process work.
“Our original motivation was to solve this problem by building a machine that could continuously twist any two materials with respect to one another, readily producing an infinite range of novel materials,” says team leader Prof. Shahal Ilani. “However, while building this machine, we discovered that it can also be turned into a very powerful microscope, capable of seeing quantum electronic waves in ways that were unimaginable before.”
Prof. Ilani and his group teamed up with their departmental colleagues Profs. Ady Stern, Binghai Yan, Yuval Oreg, and Erez Berg to explore the capabilities of the QTM, which capitalizes on a striking aspect of the quantum world: that particles (i.e., electrons) exist as both matter and energy waves, and thus can exist in many places at once. The QTM can visualize quantum electronic waves directly, giving the researchers a way to reveal the quantum activity inside a given material.
The scientists replaced the super-sharp tip of a microscope used for imaging surfaces at the atomic level with a tip that contains a flat layer of quantum material, such as a single layer of graphene. When this layer is brought into contact with the surface of the material of interest, it forms a two-dimensional interface across which electrons can tunnel at many different locations. Quantum mechanically, they tunnel in all locations simultaneously, and the tunneling events at different locations interfere with each other. This interference allows an electron to tunnel only if its wave functions on both sides of the interface match exactly.
Generally, the waves in the tip and the sample propagate in different directions and therefore do not match. The QTM uses its twisting capability to find the angle at which matching occurs: By continuously twisting the tip with respect to the sample, the tool causes their corresponding wave functions to also twist with respect to one another. Once these wave functions match on both sides of the interface, tunneling can occur—and the QTM can map the position. Knowing at which angles electrons cross the interface supplies the researchers with a great deal of information about the probed material.
The Weizmann team has already applied their microscope to studying the properties of several key quantum materials at room temperature and now plans to conduct experiments at ultracold temperatures, where some of the most exciting quantum mechanical effects occur.
The ability to peer so deeply into the quantum world can help reveal fundamental truths about nature and may have a tremendous effect on emerging technologies.
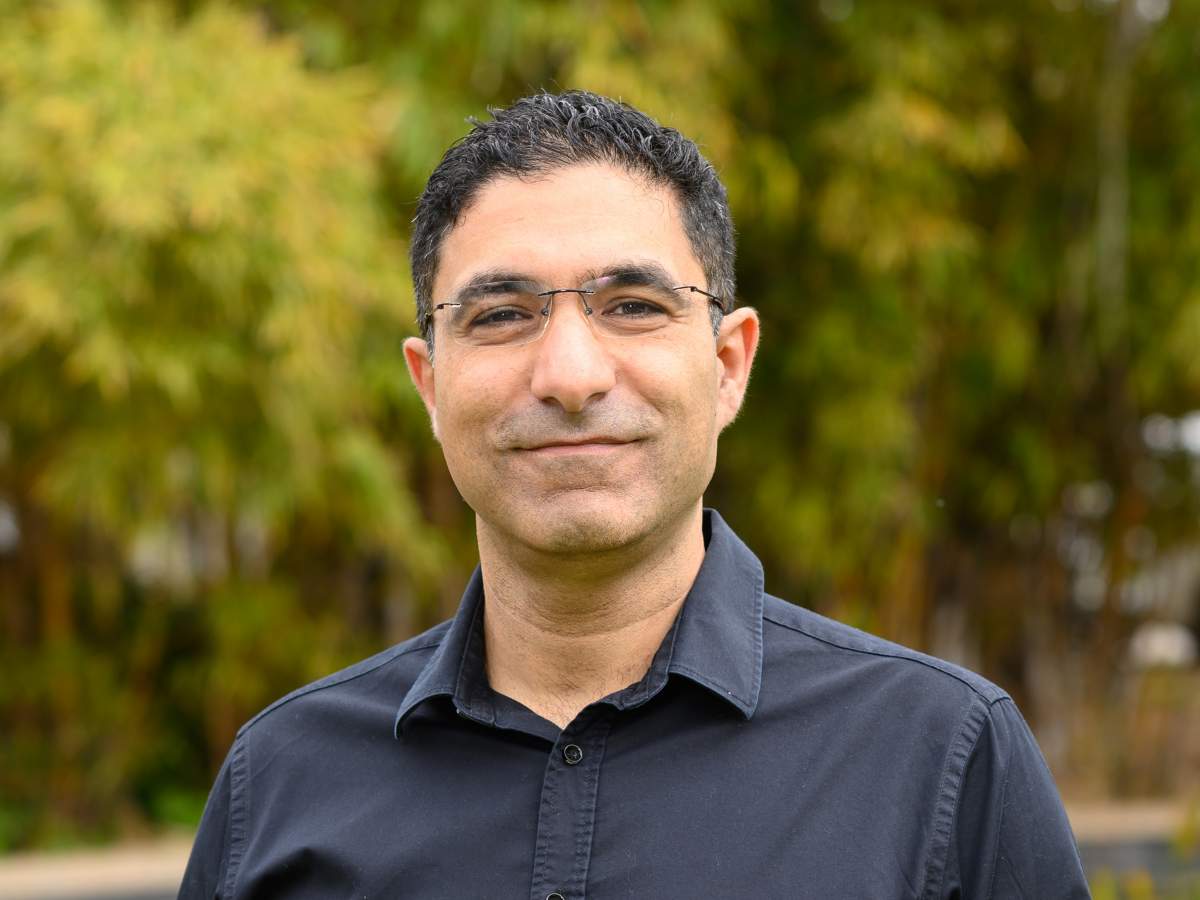
Prof. Shahal Ilani’s research is supported by the Helen and Martin Kimmel Award for Innovative Investigation, the Sagol Weizmann-MIT Bridge Program, and the Rosa and Emilio Segre Research Award.
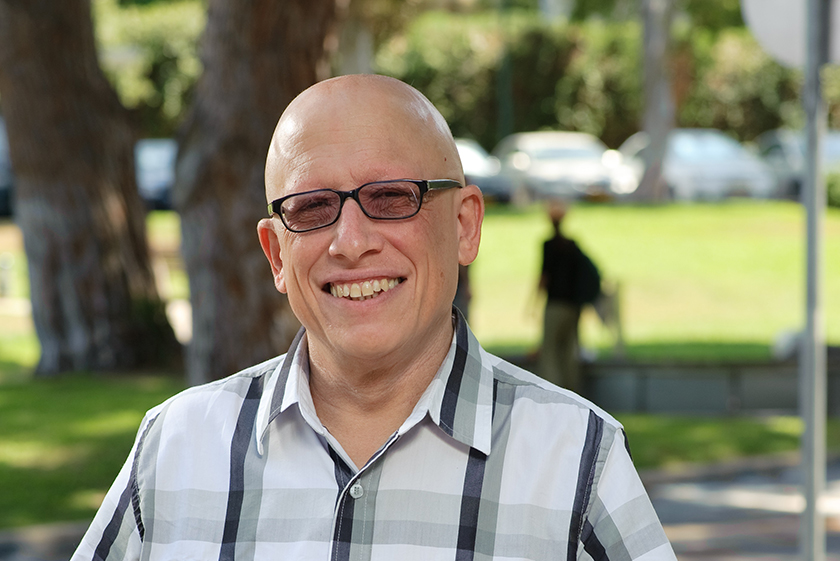
Prof. Ady Stern is Head of the Schwartz Reisman Institute for Theoretical Physics.
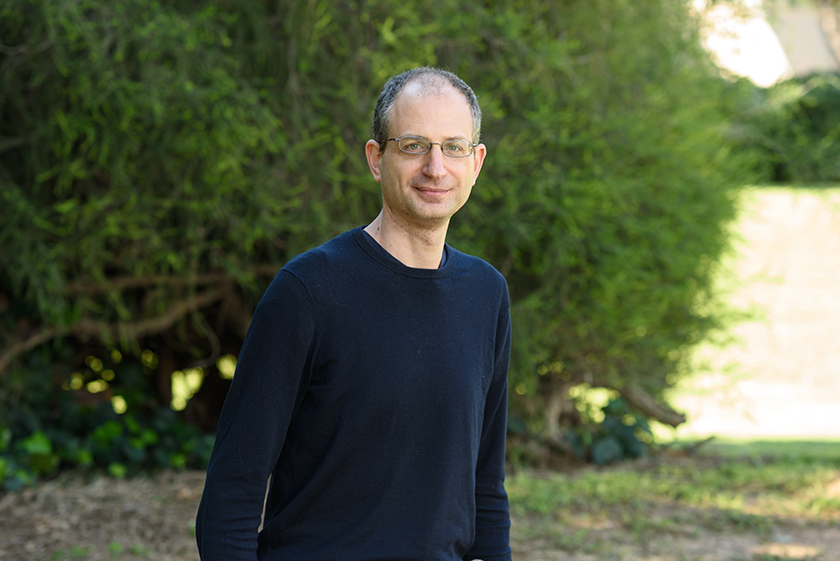
Prof. Erez Berg.